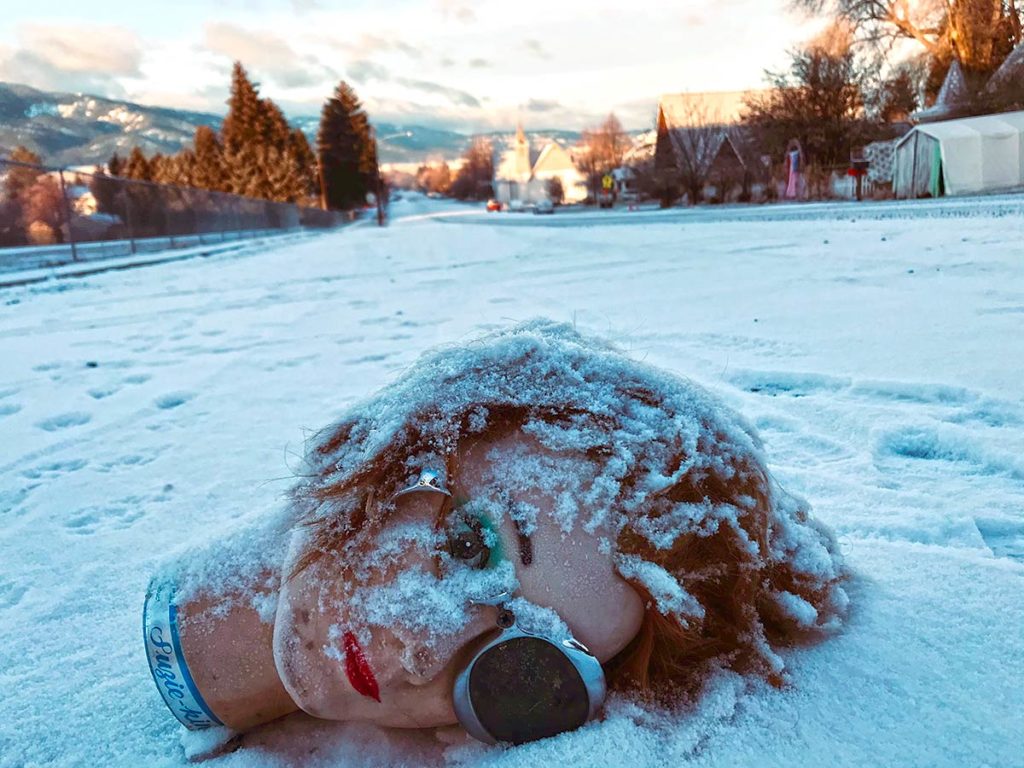
I have few more pungent olfactory memories than those from the late summers of my childhood. The grapes growing softer and sweeter, the smell of the grasses changing from green and sappy to spicy, sweet and dry, the crackling of insect noises in the fields and forests. But nothing could compare to the purple-black, heady waves of tempting aromas that tumbled from the bramble patches on the farm.
Seeing the first ripe blackberry of the season sparked the beginning of heroic efforts to eat as many of the tender, shiny jewels as possible before they lost their turgid bounce, grew cloyingly sweet and seedy, and fed the birds and other hungry beasts took their piece with their autumnal appetites driving the frenzy of late summer binges to prepare for the winter. But those weeks were ripe with my aunt’s pies, tarts, jams and as many hands full of just pure, unadulterated summer-in-your-mouth delight as possible.
Of course there’s always a catch. I have permanent scars from the recurved harpoons that grab you just as your fingers brush up against the perfect fat berry that is peeking out just so, hooking the outer dermis of hand, forearm, scalp as you gingerly try to extract the little gem. But ask any bird, bug or kid why they suffer the piercing prickles and the answer is unanimous, “It’s worth it”.
It wasn’t until I was older that my high school biology teacher introduced me to the terms ‘invasive,’ ‘exotic,’ and ‘noxious,’ weeds. They squeeze out native, even endemic species, reducing biodiversity, changing soil conditions, smothering, choking, changing. I was radicalized, naturally, and participated eagerly in graduate seminars and workshops focused on words like ‘eradicate’ and ‘control,’ our natural areas at risk of complete and permanent destruction. The tools and weapons employed in this war are legion, and as a devoted soldier I followed the directives of the literature on invasive species, growing more and more alarmed as our tactics clearly failed, the war effort growing ever more sisyphian, demoralizing, and painful.
It was when I left public lands and came back to farmland that I allowed myself to reexamine many of the key tenets of my ecological education: what if our perspective on biodiversity, natives and these demons we call invasive is rooted in a weak understanding or at least a blindness to two foundational concepts: succession and selection pressure? Meta-analyses of the research on and strategies for controlling invasive species reveal an impressive expenditure of effort and resources on a litany of attempts world-wide (see the bibliography below), but I challenge anyone to point to the resounding success that any of these efforts has yielded. We have spared no expense, no mechanical or chemical warfare, in this effort. And we cannot even boast of battles we’ve won let alone the war.
What if we have fundamentally missed what this chronic decline is telling us? What if invasives are the symptom, not the disease?
—
Since the first simple organisms left the oceans and did the first work of using atmospheric gas to turn rock into soil, natural selection and evolution have met the challenges of the physical non-living world by shaping it with life. Complex life only exists because of the billions of years spent by living organisms painstakingly making the substrate for future, more complex life to thrive.
As bacteria and fungi and protozoa and rotifer evolved, so did their food and support plants, shaping new environments for even further below-ground diversity. That diversity facilitated an environment suitable for greater plant diversity, and the greater plant diversity created conditions that supported greater diversity in the animal kingdom, and so on.
In nature, bare ground signifies the kind of natural disturbance that happens only occasionally across geological time, not in human time: volcanic eruptions, earthquakes, asteroids. Bare soil is a fire alarm. Bare ground is the equivalent of an arterial bleed. At the species level, plants and animals have coevolved relationships with one another that allow them to create the conditions necessary to recover bare ground back to a baseline of health and function after a disturbance. There is NO reference point in the history of our world that supports the idea that life gets less diverse permanently. It contracts, and there are powerful examples of massive extinction events, now rivaled by this one we are in right now, but in every instance the rebuilding life always radiates towards more diversity.
Yet the Anthropocene, if it is anything, is the age of bare ground on purpose. Every time we use the plow, we reset the system to a primary stage.
What happens when land is repeatedly stripped down to bare earth? Edges of fragility radiate from the wound. As we do this repeatedly, and convert more and more acres from native habitat to cultivated, controlled farmland, this bleed out grows larger and larger. Eventually, the connective tissue can no longer support the more sensitive plants and animals, the very communities that sustained it before.
The scope and severity of the disturbance pattern we have created has become such that our ‘healthy’ ecosystems are moving backwards, successionally. We keep losing diversity in places that are supposed to be protected, because the edges represent more vulnerable surface area than is protected within.
Human-based disturbance patterns: converting the land, removing the native vegetation, artificially draining the land, repeatedly tilling and plowing; this pattern has created a landing pad for a specific type of organism. Many species we call ‘invasive’ have evolutionary strategies that make them brilliant colonizers of disturbed land: lightweight seeds, delicious fruits, vegetative (clonal) reproduction, rapid growth, low nutrient requirements, defense mechanisms adapted to harsh environments, and so much more. These organisms succeed because they have the stuff to thrive in places where our actions on the landscape have pushed ecosystems into suspended primary stages. The diversity of native species we want to see instead of these invasives requires a stability we cannot even see or comprehend that we have lost.
Quorum sensing is a particularly cool and well-studied mechanism in biology. Essentially, it is the process whereby microbes use various signaling pathways to communicate and ‘discern’ population density, in order to regulate a behavior or even express a trait like virulence or the formation of biofilms. In other words, they might just hang out doing nothing until a critical mass can achieve a particular effort, and then leap into action. This has been studied widely in bacteria and fungi. My point? It is not so far-fetched, given the many ways we know species sense, communicate, and react to one another, that ecosystems would ‘sit’ in a particular state until the necessary components have recovered to a point where the community can, through cooperative processes, facilitate forward progress again.
—
Every ecosystem is, in its way, an organism. Critical processes within the organism require complex sets of locks and their keys in order to take place. In the ecosystem of our bodies, all of the most critical functions that keep us well and healthy, from respiration to reproduction to neuronal communication and DNA repair, require enzymatic reactions catalyzed by trace minerals and vitamins, without which, these processes are stalled. We are now in an age where the sources of those nutrients, the foods we eat, no longer contain them in the quantities they once did. We are deprived, even if we eat more. The ecosystem can function without some of its pieces for a time, but eventually, the parts cannot communicate, and the components decay and decline, contracting down to whatever unit can survive until better conditions arrive.
How we approach and care for our ecosystems has many parallels with how we approach our health. We are masters of acute care. We can set bones, sew and repair even morbid wounds. But chronic modern diseases have exposed the limits of acute care, just as invasive species have exposed our ecological blind spots. Intelligent societies might by now realize that healthy, functioning ecosystems are the only basis for the survival of future generations.
—————
If our objective is to maintain high functioning ecosystems and protect biodiversity, and our strategy is to eradicate (read: more disturbance) or control invasive weeds, towards which we employ tactics focused solely on the offending vegetation and possibly revegetation with native stock, we are likely to continue to fail for several reasons. Our tactics are addressing the superficial presentation, the symptom, not the root cause. Deeply wounded are our systems. The native plants we seed and plug cannot be fit without their microbiological partners, the vertebrate and invertebrate herbivores that stimulate their metabolism to produce phytochemicals for their protection, that pollinate their flowers and spread their seeds. The objective is good, but the strategy and tactics are misguided.
It takes non-human time to move from a primary or early successional stage to something that is at once stable and dynamic. Soil food webs are not quaint pictures. Soil food webs are critical mortar for anything more ‘macro’. Do we know what the geospatial requirements are for an ecosystem to have the means to function well? We do not, but we DO know that highly disturbed, bare ground is fertile ground for certain organisms that have evolved strategies that make them highly competitive when conditions are poor. Our efforts could potentially do more harm than good if we manage for symptoms.
What if we changed our strategy? If our objective is high-functioning ecosystems, both on the landscape and in our bodies, that repair quickly, respond quickly, maintain good productivity, nutrient cycling and energetic exchange, how would we identify a strategy to reach that objective? Could the strategy be to look first at the biggest threat to those functions, which is AGRICULTURE as we practice it currently?
—-
Since you’re still reading, indulge me in a thought experiment. Imagine we focus our incredible potential, our power and resources, on first the most basic support mechanisms to create the conditions required for greater resilience and more stable diversity. Imagine that an agricultural region could muster a majority of privately held lands and landowners with the goal of adopting certain practices that support flows, the shape of which should be circles, not lines. We would certainly start with soil and nutrient cycling. However, by including thoughtfully designed installations of habitat networks on farmland, that are connected across time and property line, a deeper, more architectural repair of the soil can facilitate a deeper, more architectural stability above ground.
This return of lost trophic structure is key to an ecological engine that revs, that cycles times faster. And if we could also come together as farmers, with the support of our communities and governments, to plan crop rotations that feed, build and fuel locally, we no longer export our fertility beyond our borders, we feed more with less waste, and farmers feel secure in their work again without the pressure of squeezing one more penny out of a commodity market that doesn’t know or care about them.
This kind of planning and design could and should bring together a rich and diverse community of people who love where they live. It could bring artistry to the landscape, generating more peaceful and tranquil places for people to visit and recreate. It could allow for greater connection between public and private lands, each shoring up the other, making more space for wildlife and generating more public support of landowners who participate.
Once more let us consider the herculean discharge of energy in the effort to ‘eradicate’ a particular Outcast species, while we blindly eliminate legions of endangered species every year. The symptoms we dislike, the thorny, prickly, aggressive species we focus on are doing a job. They are not the cause, they are the symptomatic expression of a human disturbance regime that we impose repeatedly, in human time, that has geologic time level impact. If we look for tactics that recharge the battery that our plant communities plug into, we might just be able to see something happen in human time that looks more like succes
—-
Resources, and for further reading:
SUCCESSION
Bauer, J. T., Mack, K. M. L., & Bever, J. D. (2015). Plant‐soil feedbacks as drivers of succession: Evidence from remnant and restored tallgrass prairies. Ecosphere, 6, art158.
Bever, J. D., Platt, T. G., & Morton, E. R. (2012). Microbial population and community dynamics on plant roots and their feedbacks on plant communities. Annual Review of Microbiology, 66, 265– 283.
Blois, J. L., Zarnetske, P. L., Fitzpatrick, M. C., & Finnegan, S. (2013). Climate change and the past, present, and future of biotic interactions. Science, 341, 499– 504.
Chai, Y., Liu, X., Yue, M., Guo, J., Wang, M., Wan, P., … Zhang, C. (2015). Leaf traits in dominant species from different secondary successional stages of deciduous forest on the Loess Plateau of northern China. Applied Vegetation Science, 18, 50– 63.
Chang, C. C., Halpern, C. B., Antos, J. A., Avolio, M. L., Biswas, A., Cook, J. E., … Zobel, D. B. (2019). Testing conceptual models of early plant succession across a disturbance gradient. Journal of Ecology, 107, 517– 530.
Chang, CC, Turner, BL. Ecological succession in a changing world. J Ecol. 2019; 107: 503– 509. https://doi.org/10.1111/1365-2745.13132
Clark, A. T., Knops, J. M. H., & Tilman, D. (2019). Contingent factors explain average divergence in functional composition over 88 years of old field succession. Journal of Ecology, 107, 545– 558.
Connell, J. H., & Slatyer, R. O. (1977). Mechanisms of succession in natural communities and their role in community stability and organization. The American Naturalist, 111, 1119– 1144.
Cutler, N. A., Chaput, D. L., & van der Gast, C. J. (2014). Long‐term changes in soil microbial communities during primary succession. Soil Biology and Biochemistry, 69, 359– 370.
Damschen, E. I., Brudvig, L. A., Haddad, N. M., Levey, D. J., Orrock, J. L., & Tewksbury, J. J. (2008). The movement ecology and dynamics of plant communities in fragmented landscapes. Proceedings of the National Academy of Sciences of the United States of America, 105, 19078– 19083.
De Meester, L., Vanoverbeke, J., Kilsdonk, L. J., & Urban, M. C. (2016). Evolving perspectives on monopolization and priority effects. Trends in Ecology & Evolution, 31, 136– 146.
Derroire, G., Powers, J. S., Hulshof, C. M., Varela, L. E. C., & Healey, J. R. (2018). Contrasting patterns of leaf trait variation among and within species during tropical dry forest succession in Costa Rica. Scientific Reports, 8, 285.
Diaz, S., & Cabido, M. (2001). Vive la difference: Plant functional diversity matters to ecosystem processes. Trends in Ecology & Evolution, 16, 646– 655.
Dickie, I. A., Koide, R. T., & Fayish, A. C. (2001). Vesicular–arbuscular mycorrhizal infection of Quercus rubra seedlings. New Phytologist, 151, 257– 264.
Dini‐Andreote, F., Stegen, J. C., van Elsas, J. D., & Salles, J. F. (2015). Disentangling mechanisms that mediate the balance between stochastic and deterministic processes in microbial succession. Proceedings of the National Academy of Sciences of the United States of America, 112, E1326– E1332.
Douma, J. C., de Haan, M. W. A., Aerts, R., Witte, J.‐P. M., & van Bodegom, P. M. (2012). Succession‐induced trait shifts across a wide range of NW European ecosystems are driven by light and modulated by initial abiotic conditions. Journal of Ecology, 100, 366– 380.
Duffin, K. I., Li, S. P., & Meiners, S. J. (2019). Species pools and differential performance generate variation in leaf nutrients between native and exotic species in succession. Journal of Ecology, 107, 595– 605.
Egerton, F. N. (2015). History of ecological sciences, part 54: Succession, community, and continuum. The Bulletin of the Ecological Society of America, 96, 426– 474.
Ettinger, A. K., & HilleRisLambers, J. (2013). Climate isn’t everything: Competitive interactions and variation by life stage will also affect range shifts in a warming world. American Journal of Botany, 100, 1344– 1355.
Fischer, D. G., Antos, J. A., Biswas, A., & Zobel, D. B. (2019). Understorey succession after burial by tephra from Mount St. Helens. Journal of Ecology, 107, 531– 544.
Fridley, J., & Wright, J. (2012). Drivers of secondary succession rates across temperate latitudes of the Eastern USA: Climate, soils, and species pools. Oecologia, 168, 1069– 1077.
Fukami, T. (2015). Historical contingency in community assembly: Integrating niches, species pools, and priority effects. Annual Review of Ecology, Evolution, and Systematics, 46, 1– 23.
Gao, C., Montoya, L., Xu, L., Madera, M., Hollingsworth, J., Purdom, E., … Lemaux, P. G. (2018). Strong succession in arbuscular mycorrhizal fungal communities. The ISME Journal, 1, 214– 226.
Garnier, E., Cortez, J., Billes, G., Navas, M. L., Roumet, C., Debussche, M., … Toussaint, J. P. (2004). Plant functional markers capture ecosystem properties during secondary succession. Ecology, 85, 2630– 2637.
Hall, F., Bryant, L., Clausnitzer, R., Geier-Hayes, K., Keane, R., Kertis, J., Shlisky, A., Steele, R., Definitions and Codes for Seral Status and Structure of Vegetation USFS Publication
Härdtle, W., Niemeyer, M., Niemeyer, T., Assmann, T., & Fottner, S. (2006). Can management compensate for atmospheric nutrient deposition in heathland ecosystems? Journal of Applied Ecology, 43, 759– 769.
HilleRisLambers, J., Adler, P. B., Harpole, W. S., Levine, J. M., & Mayfield, M. M. (2012). Rethinking community assembly through the lens of coexistence theory. Annual Review of Ecology, Evolution, and Systematics, 43, 227– 248.
Huston, M., & Smith, T. (1987). Plant succession: Life history and competition. The American Naturalist, 130, 168– 198.
Jangid, K., Whitman, W. B., Condron, L. M., Turner, B. L., & Williams, M. A. (2013). Soil bacterial community succession during long‐term ecosystem development. Molecular Ecology, 22, 3415– 3424.
Johnson, N. C., Zak, D. R., Tilman, D., & Pfleger, F. L. (1991). Dynamics of vesicular‐arbuscular mycorrhizae during old field succession. Oecologia, 86, 349– 358.
Jones, C. C., & del Moral, R. (2009). Dispersal and establishment both limit colonization during primary succession on a glacier foreland. Plant Ecology, 204, 217– 230.
Kahmen, S., & Poschlod, P. (2004). Plant functional trait responses to grassland succession over 25 years. Journal of Vegetation Science, 15, 21– 32.
Karadimou, E., Kallimanis, A., Tsiripidis, I., Raus, T., Bergmeier, E., & Dimopoulos, P. (2018). Functional diversity changes over 100 yr of primary succession on a volcanic island: Insights into assembly processes. Ecosphere, 9, e02374.
Kelemen, A., Tóthmérész, B., Valkó, O., Miglécz, T., Deák, B., & Török, P. (2017). New aspects of grassland recovery in old‐fields revealed by trait‐based analyses of perennial‐crop‐mediated succession. Ecology and Evolution, 7, 2432– 2440.
Kettenring, K.M. and Adams, C.R. (2011), Lessons learned from invasive plant control experiments: a systematic review and meta‐analysis. Journal of Applied Ecology, 48: 970-979. doi:10.1111/j.1365-2664.2011.01979.x
Koziol, L., & Bever, J. D. (2019). Mycorrhizal feedbacks generate positive frequency dependence accelerating grassland succession. Journal of Ecology, 107, 622- 632.
Kraft, N. J. B., Adler, P. B., Godoy, O., James, E. C., Fuller, S., & Levine, J. M. (2014). Community assembly, coexistence and the environmental filtering metaphor. Functional Ecology, 29, 592– 599.
Lai, H., Chong, K., Yee, A., Tan, H., & van Breugel, M. (in revision). Functional traits that moderate tropical plant recruitment during post-windstorm secondary succession.
Lambers, H., Raven, J. A., Shaver, G. R., & Smith, S. E. (2008). Plant nutrient‐acquisition strategies change with soil age. Trends in Ecology & Evolution, 23, 95– 103.
Lasky, J. R., Uriarte, M., Boukili, V. K., & Chazdon, R. L. (2014). Trait‐mediated assembly processes predict successional changes in community diversity of tropical forests. Proceedings of the National Academy of Sciences of the United States of America, 111, 5616– 5621.
Lebrija‐Trejos, E., Perez‐Garcia, E. A., Meave, J. A., Bongers, F., & Poorter, L. (2010). Functional traits and environmental filtering drive community assembly in a species‐rich tropical system. Ecology, 91, 386– 398.
Li, S., Cadotte, M. W., Meiners, S. J., Pu, Z., Fukami, T., & Jiang, L. (2016). Convergence and divergence in a long‐term old‐field succession: The importance of spatial scale and species abundance. Ecology Letters, 19, 1101– 1109.
Liu, J., Coomes, D. A., Hu, G., Liu, J., Yu, J., Luo, Y., & Yu, M. (2019). Larger fragments have more late‐successional species of woody plants than smaller fragments after 50 years of secondary succession. Journal of Ecology, 107, 582– 594.
Lohbeck, M., Poorter, L., Martínez‐Ramos, M., Rodriguez‐Velázquez, J., van Breugel, M., & Bongers, F. (2014). Changing drivers of species dominance during tropical forest succession. Functional Ecology, 28, 1052– 1058.
Makoto, K., & Wilson, S. D. (2016). New multicentury evidence for dispersal limitation during primary succession. The American Naturalist, 187, 804– 811.
Makoto, K., & Wilson, S. D. (2019). When and where does dispersal limitation matter in primary succession? Journal of Ecology, 107, 559– 565.
Marteinsdóttir, B., Svavarsdóttir, K., & Thórhallsdóttir, T. E. (2018). Multiple mechanisms of early plant community assembly with stochasticity driving the process. Ecology, 99, 91– 102.
McGuire, K., Henkel, T., de la Cerda, I. G., Villa, G., Edmund, F., & Andrew, C. (2008). Dual mycorrhizal colonization of forest‐dominating tropical trees and the mycorrhizal status of non‐dominant tree and liana species. Mycorrhiza, 18, 217– 222.
Meiners, S. J., Cadotte, M. W., Fridley, J. D., Pickett, S. T. A., & Walker, L. R. (2014). Is successional research nearing its climax? New approaches for understanding dynamic communities. Functional Ecology, 29, 154– 164.
Norden, N., Angarita, H. A., Bongers, F., Martínez‐Ramos, M., Granzow‐de la Cerda, I., van Breugel, M., … Chazdon, R. L. (2015). Successional dynamics in Neotropical forests are as uncertain as they are predictable. Proceedings of the National Academy of Sciences of the United States of America, 112, 8013– 8018.
Peltzer, D. A., Wardle, D. A., Allison, V. J., Baisden, W. T., Bardgett, R. D., Chadwick, O. A., … Richardson, S. J. (2010). Understanding ecosystem retrogression. Ecological Monographs, 80, 509– 529.
Prach, K., Tichý, L., Lencová, K., Adámek, M., Koutecký, T., Sádlo, J., … Botta‐Dukát, Z. (2016). Does succession run towards potential natural vegetation? An analysis across seres. Journal of Vegetation Science, 27, 515– 523.
Prach, K., & Walker, L. R. (2011). Four opportunities for studies of ecological succession. Trends in Ecology & Evolution, 26, 119– 123.
Prach, K., & Walker, L. R. (2019). Differences between primary and secondary plant succession among biomes of the world. Journal of Ecology, 107, 510– 516.
Pulsford, S. A., Lindenmayer, D. B., & Driscoll, D. A. (2014). A succession of theories: Purging redundancy from disturbance theory. Biological Reviews, 91, 148– 167.
Purschke, O., Schmid, B. C., Sykes, M. T., Poschlod, P., Michalski, S. G., Durka, W., … Prentice, H. C.(2013). Contrasting changes in taxonomic, phylogenetic and functional diversity during a long‐term succession: Insights into assembly processes. Journal of Ecology, 101, 857– 866.
Reich, P. B. (2014). The world‐wide ‘fast–slow’ plant economics spectrum: A traits manifesto. Journal of Ecology, 102, 275– 301.
Suding, K. N., & Hobbs, R. J. (2009). Threshold models in restoration and conservation: A developing framework. Trends in Ecology & Evolution, 24, 271– 279.
Tarlera, S., Jangid, K., Ivester, A. H., Whitman, W. B., & Williams, M. A. (2008). Microbial community succession and bacterial diversity in soils during 77000 years of ecosystem development. FEMS Microbiology Ecology, 64, 129– 140.
Teste, F. P., & Laliberté, E. (2019). Plasticity in root symbioses following shifts in soil nutrient availability during long‐term ecosystem development. Journal of Ecology, 107, 633– 649.
Tilman, D. (1993). Community diversity and succession – The roles of competition, dispersal, and habitat modification. Biodiversity and Ecosystem Function, 99, 327– 344.
Uroz, S., Tech, J., Sawaya, N., Frey‐Klett, P., & Leveau, J. (2014). Structure and function of bacterial communities in ageing soils: Insights from the Mendocino ecological staircase. Soil Biology and Biochemistry, 69, 265– 274.
Walker, L. R. (2011). Integration of the study of natural and anthropogenic disturbances using severity gradients. Austral Ecology, 36, 916– 922.
Walker, L. R., & del Moral, R. (2003). Primary succession and ecosystem rehabilitation. Cambridge, UK: Cambridge University Press.
Walker, L. R., & del Moral, R. (2009). Lessons from primary succession for restoration of severely damaged habitats. Applied Vegetation Science, 12, 55– 67.
Walker, L. R., Walker, J., & Hobbs, R. J. (2007). Linking restoration and ecological succession. London, UK: Springer.
Walker, L. R., & Wardle, D. A. (2014). Plant succession as an integrator of contrasting ecological time scales. Trends in Ecology & Evolution, 29, 504– 510.
Wardle, D. A., Walker, L. R., & Bardgett, R. D. (2004). Ecosystem properties and forest decline in contrasting long‐term chronosequences. Science, 305, 509– 513.
Wright, S. J., Kitajima, K., Kraft, N. J., Reich, P. B., Wright, I. J., Bunker, D. E., … Díaz, S. (2010). Functional traits and the growth–mortality trade‐off in tropical trees. Ecology, 91, 3664– 3674.
Wright, I. J., Reich, P. B., Westoby, M., Ackerly, D. D., Baruch, Z., Bongers, F., … Diemer, M. (2004). The worldwide leaf economics spectrum. Nature, 428, 821– 827.
Zemunik, G., Turner, B. L., Lambers, H., & Laliberté, E. (2015). Diversity of plant nutrient‐acquisition strategies increases during long‐term ecosystem development. Nature Plants, 1, 15050. https://doi.org/10.1038/nplants.2015.50
ON INVASIVE SPECIES, THEIR STUDY AND CONTROL:
Felker-Quinn, E., Schweitzer, J., Bailey, J., (2013). Meta-analysis reveals evolution in invasive plant species but little support for Evolution of Increased Competitive Ability (EICA) Ecology and Evolution 3(3): 739-751
Abhilasha, D., and J. Joshi. 2009. Enhanced fitness due to higher fecundity, increased defence against a specialist and tolerance towards a generalist herbivore in an invasive annual plant. J. Plant Ecol. UK 2:77–86.
Agrawal, A. A., A. P. Hastings, M. T. J. Johnson, J. L. Maron, and J.‐P. Salminen. 2012. Insect Herbivores Drive Real‐Time Ecological and Evolutionary Change in Plant Populations. Science 338:113–116.
Alexander, J. M., P. J. Edwards, M. Poll, C. G. Parks, and H. Dietz. 2009. Establishment of parallel altitudinal clines in traits of native and introduced forbs. Ecology 90:612–622.
Allan, E., and J. R. Pannell. 2009. Rapid divergence in physiological and life‐history traits between northern and southern populations of the British introduced neo‐species, Senecio squalidus. Oikos118:1053–1061.
Alvarez, N., C. Thiel‐Egenter, A. Tribsch, R. Holderegger, S. Manel, P. Schoenswetter, et al. 2009. History or ecology? Substrate type as a major driver of patial genetic structure in Alpine plants Ecol. Lett. 12:632–640.
Barto, E. K., and M. C. Rillig. 2012. Dissemination biases in ecology: effect sizes matter more than quality. Oikos 121:228–235.
Beaumont, M. A., and D. J. Balding. 2004. Identifying adaptive genetic divergence among populations from genome scans. Mol. Ecol. 13:969–980.
Blair, A. C., and L. M. Wolfe. 2004. The evolution of an invasive plant: An experimental study with Silene latifolia. Ecology 85:3035–3042.
Blossey, B., and R. Nötzold. 1995. Evolution of increased competitive ability in invasive nonindigenous plants: a hypothesis. J. Ecol.83:3.
Blumenthal, D. M., and R. A. Hufbauer. 2007. Increased plant size in exotic populations: a common‐garden test with 14 invasive species. Ecology 88:2758–2765.
Bossdorf, O., D. Prati, H. Auge, and B. Schmid. 2004. Reduced competitive ability in an invasive plant. Ecol. Lett. 7:346–353.
Bossdorf, O., H. Auge, L. Lafuma, W. E. Rogers, E. Siemann, and D. Prati. 2005. Phenotypic and genetic differentiation between native and introduced plant populations. Oecologia144:1–11.
Bossdorf, O., A. Lipowsky, and D. Prati. 2008. Selection of preadapted populations allowed Senecio inaequidens to invade Central Europe. Divers. Distrib. 14:676–685.
Buschmann, H., P. J. Edwards, and H. Dietz. 2005. Variation in growth pattern and response to slug damage among native and invasive provenances of four perennial Brassicaceae species. J. Ecol. 93:322–334.
Buswell, J. M., A. T. Moles, and S. Hartley. 2011. Is rapid evolution common in introduced plant species? J. Ecol. 99:214–224.
Callaway, R. M., W. M. Ridenour, T. Laboski, T. Weir, and J. M. Vivanco. 2005. Natural selection for resistance to the allelopathic effects of invasive plants. J. Ecol. 93:576–583.
Catford, J. A., R. Jansson, and C. Nilsson. 2009. Reducing redundancy in invasion ecology by integrating hypotheses into a single theoretical framework. Divers. Distrib.15:22–40.
Cipollini, D., J. Mbagwu, K. Barto, C. Hillstrom, and S. Enright. 2005. Expression of constitutive and inducible chemical defenses in native and invasive populations of Alliaria petiolata. J. Chem. Ecol. 31:1255–1267.
Colautti, R. I., J. L. Maron, and S. C. H. Barrett. 2009. Common garden comparisons of native and introduced plant populations: latitudinal clines can obscure evolutionary inferences. Evol. Appl. 2:187–199.
Coley, P. D., J. P. Bryant, and F. S. Chapin. 1985. Resource availability and plant antiherbivore defense. Science 230:895–899.
Conner, J. K., and D. L. Hartl. 2004. A primer of ecological genetics. Sinauer Associates, Sunderland, MA.
Cripps, M. G., H. L. Hinz, J. L. McKenney, W. J. Price, and M. Schwarzlander. 2009. No evidence for an ‘evolution of increased competitive ability’ for the invasive Lepidium draba. Basic Appl. Ecol. 10:103–112.
Doorduin, L., and K. Vrieling. 2011. A review of the phytochemical support for the shifting defence hypothesis. Phytochem. Rev.10:99–106.
Durka, W., O. Bossdorf, D. Prati, and H. Auge. 2005. Molecular evidence for multiple introductions of garlic mustard (Alliaria petiolata, Brassicaceae) to North America. Mol. Ecol. 14:1697–1706.
Ehrenfeld, J. G. 2003. Effects of exotic plant invasions on soil nutrient cycling processes. Ecosystems 6:503–523.
Eigenbrode, S. D., J. E. Andreas, M. G. Cripps, H. Ding, R. C. Biggam, and M. Schwarzlander. 2008. Induced chemical defenses in invasive plants: a case study with Cynoglossum officinale L. Biol. Invasions 10:1373–1379.
Felker‐Quinn, E., J. K. Bailey, and J. A. Schweitzer. 2011. Soil biota drive expression of genetic variation and development of population‐specific feedbacks in an invasive plant. Ecology 92:1208–1214.
Franks, S. J., P. D. Pratt, F. A. Dray, and E. L. Simms. 2008. No evolution of increased competitive ability or decreased allocation to defense in Melaleuca quinquenervia since release from natural enemies. Biol. Invasions10:455–466.
Franks, S. J., G. S. Wheeler, and C. Goodnight. 2012. Genetic variation and evolution of secondary compounds in native and introduced populations of the invasive plant Melaleuca quinquenervia. Evolution66:1398–1412.
Grotkopp, E., M. Rejmanek, and T. L. Rost. 2002. Toward a causal explanation of plant invasiveness: seedling growth and life‐history strategies of 29 pine (Pinus) species. Am. Nat.159:396–419.
Guesewell, S., G. Jakobs, and E. Weber. 2006. Native and introduced populations of Solidago gigantea differ in shoot production but not in leaf traits or litter decomposition. Funct. Ecol. 20:575–584.
Haider, S., C. Kueffer, P. J. Edwards, and J. M. Alexander. 2012. Genetically based differentiation in growth of multiple non‐native plant species along a steep environmental gradient. Oecologia 170:89–99.
Handley, R. J., T. Steinger, U. A. Treier, and H. Müller‐Schärer. 2008. Testing the evolution of increased competitive ability (EICA) hypothesis in a novel framework. Ecology89:407–417.
He, W. M., Y. L. Feng, W. M. Ridenour, G. C. Thelen, J. L. Pollock, A. Diaconu, et al. 2009. Novel weapons and invasion: biogeographic differences in the competitive effects of Centaurea maculosa and its root exudate (+/‐)‐catechin. Oecologia 159:803–815.
Hinz, H. L., and M. Schwarzlaender. 2004. Comparing invasive plants from their native and exotic range: what can we learn for biological Control? Weed Technol.18:1533–1541.
Hornoy, B., M. Tarayre, M. Herve, L. Gigord, and A. Atlan. (2011). Invasive Plants and Enemy Release: evolution of Trait Means and Trait Correlations in Ulex europaeus. PLoS ONE 6:2675
Huang, W., E. Siemann, G. S. Wheeler, J. W. Zou, J. Carrillo, and J. Q. Ding. 2010. Resource allocation to defence and growth are driven by different responses to generalist and specialist herbivory in an invasive plant. J. Ecology 98:1157–1167.
Hull‐Sanders, H. M., R. Clare, R. H. Johnson, and G. A. Meyer. 2007. Evaluation of the evolution of increased competitive ability (EICA) hypothesis: loss of defense against generalist but not specialist herbivores. J. Chem. Ecol. 33:781–799.
Integrated Taxonomic Information System. Available at: http://www.itis.gov, (accessed 15 December 2012).
Johnson, R. H., H. M. Hull‐Sanders, and G. A. Meyer. 2007. Comparison of foliar terpenes between native and invasive Solidago gigantea. Biochem. Syst. Ecol. 35:821–830.
Joshi, J., and K. Vrieling. 2005. The enemy release and EICA hypothesis revisited: incorporating the fundamental difference between specialist and generalist herbivores. Ecol. Lett. 8:704–714.
Kawakami, T., T. J. Morgan, J. B. Nippert, T. W. Ocheltree, R. Keith, P. Dhakal, et al. 2011. Natural selection drives clinal life history patterns in the perennial sunflower species, Helianthus maximiliani. Mol. Ecol.20:2318–2328.
Keller, S. R., and D. R. Taylor. 2008. History, chance and adaptation during biological invasion: separating stochastic phenotypic evolution from response to selection. Ecol. Lett. 11:852–866.
van Kleunen, M., and M. Fischer. 2008. Adaptive rather than non‐adaptive evolution of Mimulus guttatus in its invasive range. Basic Appl. Ecol. 9:213–223.
van Kleunen, M., and B. Schmid. 2003. No evidence for an evolutionary increased competitive ability in an invasive plant. Ecology 84:2816–2823.
Kollmann, J., and M. J. Banuelos. 2004. Latitudinal trends in growth and phenology of the invasive alien plant Impatiens glandulifera (Balsaminaceae). Divers. Distrib. 10:377–385.
Koricheva, J., H. Nykanen, and E. Gianoli. 2004. Meta‐analysis of trade‐offs among plant antiherbivore defenses: are plants jacks‐of‐all‐trades, masters of all? Am. Nat.163:E64–E75.
Lajeunesse, M. J. 2010. Achieving synthesis with meta‐analysis by combining and comparing all available studies. Ecology91:2561–2564.
Lankau, R. A., V. Nuzzo, G. Spyreas, and A. S. Davis. 2009. Evolutionary limits ameliorate the negative impact of an invasive plant. Proc. Natl Acad. Sci. 106:15362–15367.
Lee, C. E. 2002. Evolutionary genetics of invasive species. Trends Ecol. Evol. 17:386–391.
Lloret, F., F. Medail, G. Brundu, I. Camarda, E. Moragues, J. Rita, et al. 2005. Species attributes and invasion success by alien plants on Mediterranean islands. J. Ecol.93:512–520.
Macel, M., C. S. Lawson, S. R. Mortimer, M. Smilauerova, A. Bischoff, L. Cremieux, et al. 2007. Climate vs. soil factors in local adaptation of two common plant species. Ecology 88:424–433.
Maron, J. L., M. Vila, and J. Arnason. 2004a. Loss of enemy resistance among introduced populations of St. John’s Wort (Hypericum perforatum). Ecology 85:3243–3253.
Maron, J. L., M. Vila, R. Bommarco, S. Elmendorf, and P. Beardsley. 2004b. Rapid evolution of an invasive plant. Ecol. Monogr.74:261–280.
McDowell, S. C. L. 2002. Photosynthetic characteristics of invasive and noninvasive species of Rubus (Rosaceae). Am. J. Bot.89:1431–1438.
McKenney, J. L., M. G. Cripps, W. J. Price, H. L. Hinz, and M. Schwarzlander. 2007. No difference in competitive ability between invasive North American and native European Lepidium draba populations. Plant Ecol.193:293–303.
Meyer, G., R. Clare, and E. Weber. 2005. An experimental test of the evolution of increased competitive ability hypothesis in goldenrod, Solidago gigantea. Oecologia144:299–307.
Muller, C., and N. Martens. 2005. Testing predictions of the ‘evolution of increased competitive ability’ hypothesis for an invasive crucifer. Evol. Ecol. 19:533–550.
Müller‐Schärer, H., U. Schaffner, and T. Steinger. 2004. Evolution in invasive plants: implications for biological control. Trends Ecol. Evol. 19:417–422.
Pattison, R. R., G. Goldstein, and A. Ares. 1998. Growth, biomass allocation and photosynthesis of invasive and native Hawaiian rainforest species. Oecologia117:449–459.
Pregitzer, C., J. Bailey, S. Hart, and J. Schweitzer. 2010. Soils as agents of selection: feedbacks between plants and soils alter seedling survival and performance. Evol. Ecol.24:1045–1059.
Prentis, P. J., J. R. U. Wilson, E. E. Dormontt, D. M. Richardson, and A. J. Lowe. 2008. Adaptive evolution in invasive species. Trends Plant Sci.13:288–294.
Ridenour, W. M., J. M. Vivanco, Y. L. Feng, J. Horiuchi, and R. M. Callaway. 2008. No evidence for trade‐offs: Centaurea plants from America are better competitors and defenders. Ecol. Monogr. 78:369–386.
Rosenberg, M. S., D. C. Adams, and J. Gurevitch.(2011) Metawin Home Page. Available at: http://www.metawinsoft.com(accessed 01 July 2012).
Rosenthal, R. 1979. The file drawer problem and tolerance for null results. Psychol. Bull.86:638–641.
Schweitzer, J. A., J. K. Bailey, B. J. Rehill, G. D. Martinsen, S. C. Hart, R. L. Lindroth, et al. 2004. Genetically based trait in a dominant tree affects ecosystem processes. Ecol. Lett.7:127–134.
Smith, M. D., and A. K. Knapp. 2001. Physiological and morphological traits of exotic, invasive exotic, and native plant species in tallgrass prairie. Int. J. Plant Sci.162:785–792.
Stastny, M., U. Schaffner, and E. Elle. 2005. Do vigour of introduced populations and escape from specialist herbivores contribute to invasiveness? J. Ecol. 93:27–37.
Stinchcombe, J. R., and H. E. Hoekstra. 2007. Combining population genomics and quantitative genetics: finding the genes underlying ecologically important traits. Heredity 100:158–170.
Thebaud, C., and D. Simberloff. 2001. Are plants really larger in their introduced ranges? Am. Nat. 157:231–236.
Thomas, M. B., and A. M. Reid. 2007. Are exotic natural enemies an effective way of controlling invasive plants? Trends Ecol. Evol.22:447–453.
Thompson, J. N. 1997. Evaluating the dynamics of coevolution among geographically structured populations. Ecology 78:1619–1623.
Van Driesche, R. G., R. I. Carruthers, T. Center, M. S. Hoddle, J. Hough‐Goldstein, L. Morin, et al. 2010. Classical biological control for the protection of natural ecosystems. Biol. Control Suppl. 1:S2–S33.
Vasemagi, A. 2006. The adaptive hypothesis of clinal variation revisited: single‐locus clines as a result of spatially restricted gene flow. Genetics 173:2411–2414.
Whitney, K. D., and C. A. Gabler. 2008. Rapid evolution in introduced species, ‘invasive traits’ and recipient communities: challenges for predicting invasive potential. Divers. Distrib. 14:569–580.
Williams, J. L., H. Auge, and J. L. Maron. 2008. Different gardens, different results: native and introduced populations exhibit contrasting phenotypes across common gardens. Oecologia 157:239–248.
Willis, A. J., M. B. Thomas, and J. H. Lawton. 1999. Is the increased vigour of invasive weeds explained by a trade‐off between growth and herbivore resistance? Oecologia120:632–640.
Wilson, S. D., and D. Tilman. 1993. Plant competition and resource availability in response to disturbance and fertilization. Ecology 74:599–611.
Zangerl, A. R., and M. R. Berenbaum. 2005. Increase in toxicity of an invasive weed after reassociation with its coevolved herbivore. Proc. Natl Acad. Sci. USA 102:15529–15532.
Zhang, D. Y., and X. H. Jiang. 2006. Interactive effects of habitat productivity and herbivore pressure on the evolution of anti‐herbivore defense in invasive plant populations. J. Theor. Biol. 242:935–940.
Zou, J. W., W. E. Rogers, and E. Siemann. 2008. Increased competitive ability and herbivory tolerance in the invasive plant Sapium sebiferum. Biol. Invasions 10:291–302.